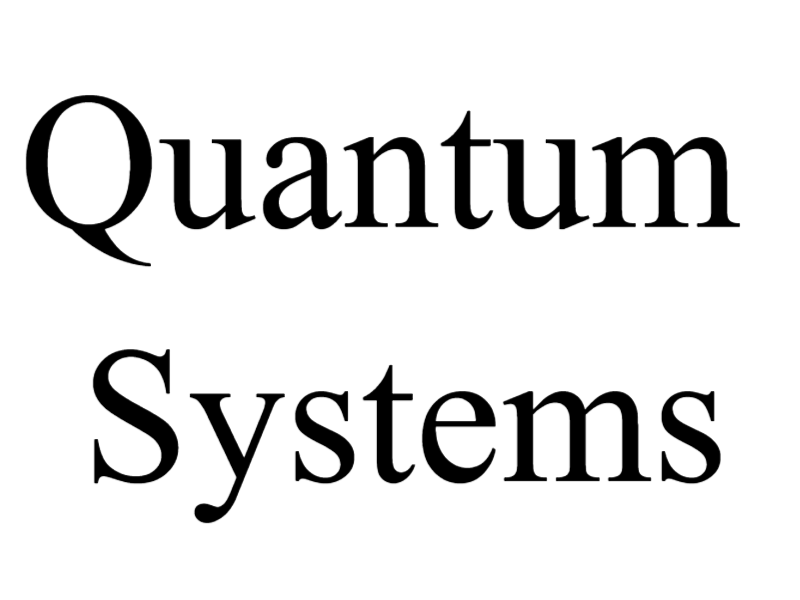
Quantum Systems: Understanding the Dynamics and Properties of Quantum Phenomena
Abstract:
This paper explores the fascinating realm of quantum systems, which form the foundation of quantum mechanics and encompass a wide range of physical systems governed by quantum phenomena. It provides an overview of the fundamental principles of quantum systems, including superposition, entanglement, and quantum coherence. The paper discusses the dynamics and properties of quantum systems, their mathematical description, and their implications for our understanding of the quantum world.
Keywords: Quantum Systems, Quantum Mechanics, Superposition, Entanglement, Quantum Coherence.
Introduction:
Quantum systems are at the heart of quantum mechanics, encompassing a diverse range of physical systems, from particles and atoms to complex quantum devices. Understanding the dynamics and properties of quantum systems is essential for unraveling the intricacies of the quantum world. This paper provides an overview of quantum systems, exploring their fundamental principles, mathematical description, and their role in our understanding of quantum phenomena.
Fundamental Principles:
Superposition: Superposition is a fundamental property of quantum systems, allowing particles to exist in multiple states simultaneously. It arises from the wave-like nature of quantum entities, enabling complex combinations of states with varying probabilities. Superposition lies at the core of quantum computations, quantum information processing, and quantum algorithms.
Entanglement: Entanglement is an intriguing property of quantum systems where particles become intrinsically correlated, regardless of distance. Entangled particles exhibit nonlocal correlations that defy classical explanations. Entanglement is crucial for quantum communication, cryptography, and the potential realization of quantum teleportation and quantum computing.
Quantum Coherence: Quantum coherence refers to the property of maintaining the phase relationships between different quantum states. It enables interference effects and allows for the creation and manipulation of delicate quantum states. Quantum coherence plays a pivotal role in applications such as quantum sensing, quantum metrology, and quantum-enhanced imaging.
Mathematical Description:
Quantum systems are described mathematically using state vectors, operators, and the principles of linear algebra. The state of a quantum system is represented by a state vector in a complex vector space, known as a Hilbert space. Operators represent physical observables, and their action on the state vector provides measurable outcomes. The time evolution of quantum systems is governed by the Schrödinger equation, which describes the dynamics of wave functions.
Dynamics and Properties:
The dynamics of quantum systems are determined by the evolution of their wave functions, governed by the Schrödinger equation. Quantum systems exhibit unique properties, including wave-particle duality, quantum interference, and the noncommutativity of observables. These properties are responsible for the fundamental differences between classical and quantum systems and give rise to the counterintuitive nature of quantum phenomena.
Implications and Applications:
Quantum systems have far-reaching implications in various fields. Quantum information processing exploits the properties of quantum systems to achieve unparalleled computational power and secure communication. Quantum sensing and metrology enable high-precision measurements beyond classical limits. Quantum simulations facilitate the study of complex quantum systems and quantum chemistry. Quantum systems also hold promise for advances in materials science, quantum optics, and quantum-inspired technologies.
Challenges and Future Perspectives:
Despite significant progress, understanding and controlling quantum systems present challenges due to issues such as decoherence, noise, and experimental limitations. Ongoing research focuses on developing methods to mitigate these challenges and improve the stability and controllability of quantum systems. The realization of scalable quantum systems and the exploration of large-scale quantum phenomena are areas of active investigation.
Conclusion:
Quantum systems lie at the heart of quantum mechanics, encompassing a wide range of physical systems governed by quantum phenomena. Understanding their dynamics and properties is essential for advancing our knowledge of the quantum world and harnessing the power of quantum technologies. Quantum systems have opened up new frontiers in computation, communication, sensing, and simulation. Continued research and technological advancements in quantum systems hold the potential to revolutionize various fields and reshape our understanding of the fundamental nature of reality.
References:
- Nielsen, M. A., & Chuang, I. L. (2010). Quantum Computation and Quantum Information. Cambridge University Press.
- Ballentine, L. E. (1998). Quantum Mechanics: A Modern Development. World Scientific.
- Sakurai, J. J., & Napolitano, J. (2017). Modern Quantum Mechanics. Cambridge University Press.
- Marquardt, F., Girvin, S. M., & Raimond, J. M. (2015). Quantum Systems: New Trends and Methods. Wiley-VCH.
- Breuer, H. P., & Petruccione, F. (2002). The Theory of Open Quantum Systems. Oxford University Press.