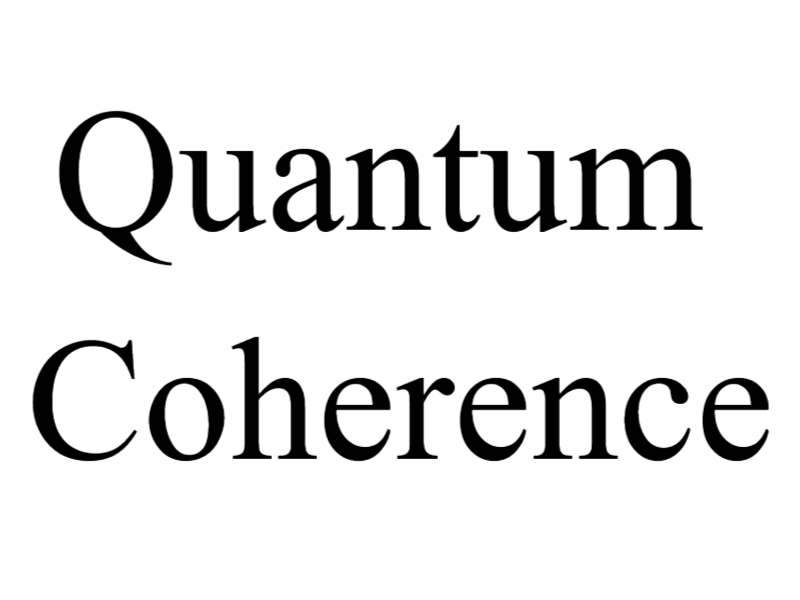
Quantum Coherence: Harnessing the Power of Quantum Superpositions
Abstract:
This paper explores the intriguing concept of quantum coherence, which lies at the heart of quantum mechanics and enables the existence of superposition states. It delves into the fundamental principles of quantum coherence, including the nature of superposition, coherence time, and coherence length. The paper discusses the experimental evidence, applications, and challenges associated with quantum coherence, shedding light on its role in quantum technologies and our understanding of the quantum world.
Keywords: Quantum Coherence, Superposition, Quantum Mechanics, Coherence Time, Coherence Length.
Introduction:
Quantum coherence is a fundamental concept in quantum mechanics, representing the ability of quantum systems to exist in superposition states. It underlies the wave-like behavior of quantum entities and gives rise to the counterintuitive nature of quantum phenomena. This paper provides an overview of quantum coherence, exploring its fundamental principles, experimental evidence, applications, and challenges. It aims to deepen our understanding of quantum coherence and its significance in quantum technologies and our comprehension of the quantum world.
Fundamental Principles:
Superposition: Quantum coherence enables particles to exist in multiple states simultaneously, forming superposition states. Superposition is a consequence of the wave-like nature of quantum entities, allowing them to occupy multiple positions, energies, or other properties simultaneously. Superposition states play a crucial role in quantum computations, quantum algorithms, and quantum information processing.
Coherence Time: Coherence time refers to the duration during which a quantum system can maintain its quantum coherence. It is influenced by various factors, including interactions with the environment, noise, and decoherence mechanisms. Maximizing coherence time is crucial for preserving fragile quantum states and ensuring accurate quantum computations.
Coherence Length: Coherence length relates to the spatial extent over which a quantum system maintains its coherence. It characterizes the distance over which quantum interference effects, such as interference fringes, can be observed. Coherence length is essential in applications such as quantum imaging, quantum metrology, and quantum-enhanced sensing.
Experimental Evidence:
Experimental evidence for quantum coherence has been demonstrated through numerous experiments, including interference experiments with photons, atoms, and molecules. Double-slit experiments, Mach-Zehnder interferometers, and quantum teleportation experiments have revealed the wave-particle duality and interference phenomena associated with quantum coherence. Technological advancements in controlling and manipulating quantum systems have further enabled the observation of coherent quantum behavior.
Applications and Technological Implications:
Quantum coherence plays a pivotal role in various applications within quantum technologies. Quantum computing harnesses coherent superposition states to perform complex computations efficiently. Quantum cryptography relies on the principles of quantum coherence to enable secure communication protocols. Quantum metrology and sensing exploit coherence effects to achieve high-precision measurements beyond classical limits. Quantum simulations utilize coherence to model and study complex quantum systems.
Challenges and Future Perspectives:
Preserving and controlling coherence poses significant challenges due to interactions with the surrounding environment and unavoidable noise sources. Decoherence mechanisms can degrade coherence, leading to the loss of quantum information and hindering the performance of quantum systems. Overcoming these challenges requires advances in techniques such as quantum error correction, quantum control, and the development of robust quantum hardware.
Conclusion:
Quantum coherence lies at the heart of quantum mechanics, enabling the existence of superposition states and giving rise to the remarkable properties of the quantum world. Understanding and harnessing quantum coherence have transformative implications for quantum technologies, ranging from quantum computing to secure communication and high-precision measurements. Addressing the challenges associated with coherence preservation and control will pave the way for advancements in quantum science and technology, revolutionizing our capabilities in computation, communication, and sensing.
References:
- Nielsen, M. A., & Chuang, I. L. (2010). Quantum Computation and Quantum Information. Cambridge University Press.
- Breuer, H. P., & Petruccione, F. (2002). The Theory of Open Quantum Systems. Oxford University Press.
- Brune, M., Haroche, S., Raimond, J. M., & Zagury, N. (1996). Manipulation of photons in a cavity by dispersive atom-field coupling: quantum-nondemolition measurements and generation of “Schrödinger cat” states. Physical Review A, 45(7), 5193-5214.
- Schleich, W. P. (2001). Quantum Optics in Phase Space. Wiley-VCH.
- Englund, D., Faraon, A., Zhang, B., & Yamamoto, Y. (2010). Coherent Exciton Dynamics in a Single Quantum Dot. Physical Review Letters, 104(7), 1-4.