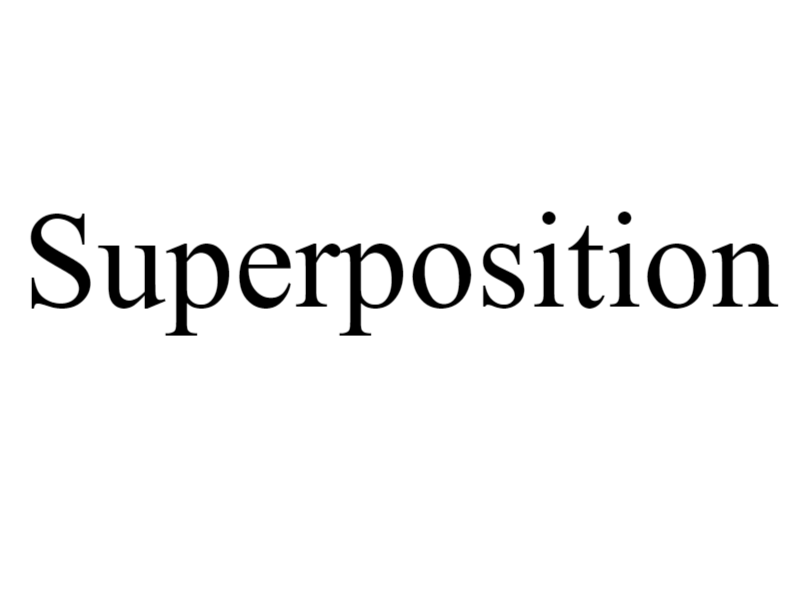
Superposition: Unveiling the Coexistence of Multiple Quantum States
Abstract:
This article explores the concept of superposition, a fundamental principle in quantum mechanics that allows particles to exist in multiple states simultaneously. It delves into the historical development of superposition, its experimental verification, and its profound implications for quantum technologies and our understanding of the quantum world.
Keywords: Superposition, Quantum Mechanics, Quantum States, Interference, Quantum Technologies.
Introduction:
Superposition is a key concept in quantum mechanics that reveals the counterintuitive behavior of particles existing in multiple states simultaneously. This principle defies classical intuition, allowing for a rich and complex description of quantum systems. This article provides an overview of superposition, tracing its historical origins, experimental evidence, and its significance in quantum technologies and scientific research.
Historical Development:
The concept of superposition arose as quantum mechanics emerged in the early 20th century. Pioneering figures such as Erwin Schrödinger and Werner Heisenberg contributed to the development of this concept. Schrödinger’s wave equation, which describes the behavior of quantum systems, introduced the idea that particles could exist in a superposition of multiple states.
Experimental Verification:
Experimental evidence has confirmed the existence of superposition. The double-slit experiment, where particles such as electrons or photons are directed at a barrier with two slits, demonstrates interference patterns that can only be explained by the simultaneous presence of multiple states. Experiments with entangled particles have also provided compelling evidence for superposition, where the measurement of one particle affects the state of the other, confirming their entangled superposition.
Superposition and Quantum Technologies:
Superposition lies at the heart of many quantum technologies. Quantum computing exploits superposition to perform computations that surpass classical capabilities. By encoding information in quantum bits or qubits, quantum computers can process vast amounts of data in parallel. Quantum cryptography harnesses superposition to ensure secure communication through quantum key distribution (QKD), utilizing the principles of quantum superposition and entanglement to enable unbreakable encryption.
Quantum Superposition and Measurement:
The principle of superposition raises questions about the role of measurement and the collapse of the wave function. Upon measurement, a quantum system’s superposition collapses into a single state, with the measurement outcome corresponding to one of the possible states. This process is governed by the probabilistic nature of quantum mechanics, where different outcomes occur with specific probabilities.
Implications and Future Directions:
Superposition has profound implications for our understanding of the quantum world and our technological capabilities. It allows for quantum systems to exhibit behaviors not seen in classical systems, enabling advancements in areas such as quantum simulations, quantum metrology, and quantum sensing. Ongoing research continues to explore the limits and applications of superposition, aiming to push the boundaries of our understanding and uncover new possibilities for quantum technologies.
Conclusion:
Superposition stands as a cornerstone of quantum mechanics, unveiling the intricate nature of quantum systems and challenging our classical intuitions. Its experimental verification and profound implications have paved the way for transformative quantum technologies and advancements in our understanding of the quantum world. As research progresses, further insights into superposition promise to unlock new frontiers in both fundamental science and practical applications.
References:
- Nielsen, M. A., & Chuang, I. L. (2010). Quantum Computation and Quantum Information. Cambridge University Press.
- Ballentine, L. E. (1998). Quantum Mechanics: A Modern Development. World Scientific.
- Feynman, R. P., Leighton, R. B., & Sands, M. (2013). The Feynman Lectures on Physics, Vol. III: The New Millennium Edition: Quantum Mechanics. Basic Books.
- Gisin, N., Ribordy, G., Tittel, W., & Zbinden, H. (2002). Quantum cryptography. Reviews of Modern Physics, 74(1), 145-195.
- Preskill, J. (2018). Quantum Computing in the NISQ Era and Beyond. Quantum, 2, 79.