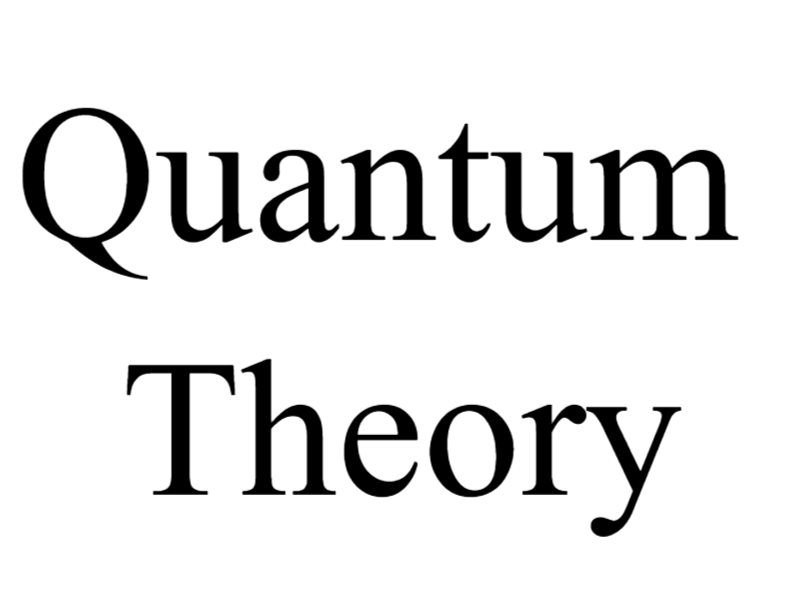
Abstract:
This paper seeks to explore Quantum Theory, its fundamental principles, the historical evolution of the theory, and its widespread impact on scientific thought and technology. In particular, it delves into the unique features of Quantum Theory, such as wave-particle duality, superposition, and entanglement, shedding light on their profound implications for understanding the physical universe. It also highlights the application of these principles in modern technology and explores potential future developments.
Keywords: Quantum Theory, Quantum Mechanics, Superposition, Entanglement, Quantum Computing.
Introduction:
Quantum Theory, the cornerstone of modern physics, presents an elegant, albeit counterintuitive, framework for understanding the behavior of matter and light at the smallest scales. Since its inception in the early 20th century, it has drastically reshaped our understanding of the physical world, challenging conventional deterministic physics and introducing a world that operates on probabilities.
Quantum Theory: A Historical Perspective:
The development of Quantum Theory was a cumulative effort of numerous scientists over several decades. Notable contributors include Max Planck, who proposed the quantum of action; Albert Einstein, who introduced the concept of photons; and Niels Bohr, who developed the Bohr model of the atom. Subsequent advancements were made by several physicists, including Werner Heisenberg, Erwin Schrödinger, and Paul Dirac, leading to the formulation of modern quantum mechanics.
Key Principles of Quantum Theory:
Quantum Theory is built around several core principles. The concept of wave-particle duality asserts that particles can display both wave-like and particle-like properties. The principle of superposition states that a quantum system can exist in multiple states simultaneously until observed. The phenomenon of entanglement allows particles to be intrinsically connected, such that the state of one instantaneously influences the other, regardless of the distance separating them.
Implications and Applications of Quantum Theory:
These quantum phenomena have vast implications, not only for our understanding of the universe but also for practical applications. Quantum mechanics has been instrumental in explaining the behavior of molecules, atoms, and subatomic particles, thereby informing areas such as chemistry, material science, and biology.
Furthermore, quantum principles are paving the way for revolutionary technologies, such as quantum computing and quantum cryptography. Quantum computers leverage superposition and entanglement to process information at scales and speeds unattainable by classical computers. Quantum cryptography utilizes quantum properties to secure information transfer, potentially making communications invulnerable to eavesdropping.
Future Prospects:
While Quantum Theory has made significant strides since its inception, many questions remain unanswered, and the full potential of quantum applications is yet to be realized. The emerging field of quantum gravity seeks to reconcile quantum mechanics with general relativity, promising a more unified understanding of the universe. The practical realization of large-scale quantum computers could revolutionize numerous sectors, including healthcare, finance, and data security.
Conclusion:
Quantum Theory, despite its counterintuitive nature, provides an invaluable framework for comprehending and manipulating the microcosm. It continues to inspire groundbreaking technological advancements that can reshape our future. As research in quantum physics progresses, the boundary of what we understand and what we can achieve expands, affirming the exciting potential held in the quantum realm.
References:
- Griffiths, D. J. (2017). Introduction to quantum mechanics. Cambridge University Press.
- Einstein, A., Podolsky, B., & Rosen, N. (1935). Can Quantum-Mechanical Description of Physical Reality Be Considered Complete?. Physical review, 47(10), 777.
- Nielsen, M. A., & Chuang, I. L. (2010). Quantum computation and quantum information: 10th anniversary edition. Cambridge University Press.
- Gisin, N., Ribordy, G., Tittel, W., & Zbinden, H. (2002). Quantum cryptography. Reviews of Modern Physics, 74(1), 145.