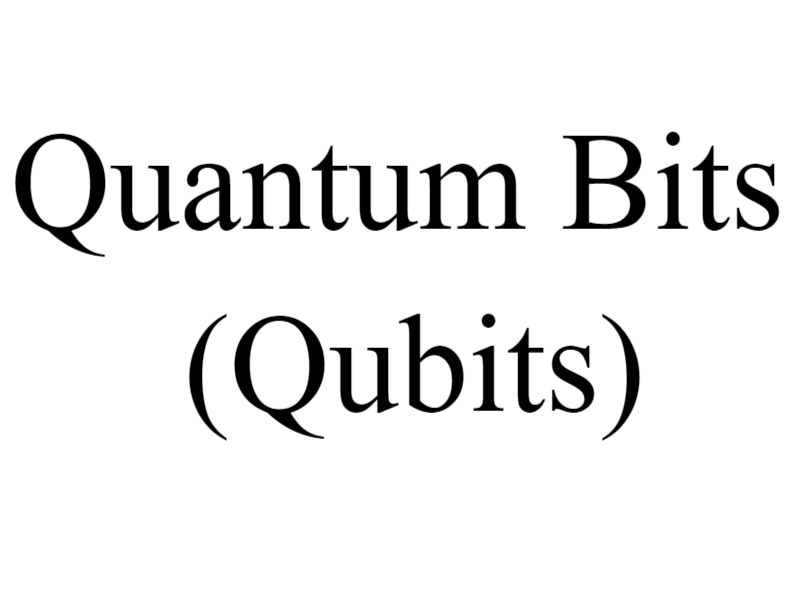
Quantum Bits (Qubits): The Building Blocks of Quantum Information
Abstract:
Quantum bits, or qubits, are the fundamental units of quantum information that lie at the heart of quantum computing and quantum information processing. This paper provides a comprehensive overview of quantum bits, including their physical realizations, mathematical representation, properties, and potential applications. We delve into the principles of superposition and entanglement that underlie qubit behavior and explore different qubit implementations, from physical systems to artificial qubits. Furthermore, we discuss the challenges and future prospects of qubits in the field of quantum technology.
Keywords: Quantum Bits, Qubits, Superposition, Entanglement, Quantum Information Processing.
Introduction:
Quantum bits, or qubits, are the foundational building blocks of quantum information processing. They differ from classical bits by harnessing the principles of quantum mechanics, allowing for the representation and manipulation of information in quantum systems. This paper aims to provide a comprehensive understanding of qubits, exploring their physical realizations, mathematical description, unique properties, and potential applications in quantum technology.
Quantum Superposition and Entanglement:
One of the key principles that differentiate qubits from classical bits is quantum superposition. Qubits can exist in multiple states simultaneously, thanks to the principle of superposition. We explore how qubits can represent a combination of 0 and 1 states, enabling parallel computations and exponential information storage. Additionally, we delve into entanglement, the phenomenon where qubits become intrinsically linked, leading to strong correlations that cannot be explained by classical physics.
Physical Realizations of Qubits:
Qubits can be physically realized in various systems, each with its advantages and challenges. We discuss prominent physical systems used as qubits, including superconducting circuits, trapped ions, topological qubits, and quantum dots. We explore the mechanisms behind encoding and manipulating qubits within these systems, highlighting their unique properties and technological advancements.
Mathematical Representation of Qubits:
Mathematically, qubits are represented using complex vectors in a two-dimensional Hilbert space. We introduce the Bloch sphere representation, which visualizes qubit states in terms of spherical coordinates. The mathematical framework allows for the manipulation of qubit states using quantum gates and quantum operations, facilitating quantum information processing tasks.
Properties of Qubits:
Qubits possess several important properties that distinguish them from classical bits. These properties include coherence, which refers to the ability of qubits to maintain their quantum states over time, and decoherence, which describes the loss of quantum coherence due to interactions with the environment. We discuss the challenges associated with preserving qubit coherence and the development of error correction techniques to mitigate errors.
Applications of Qubits:
Qubits serve as the foundation for quantum information processing, opening up new possibilities for computational algorithms, cryptography, and simulation. We explore potential applications, such as quantum simulation of complex systems, quantum cryptography protocols like quantum key distribution, and the development of quantum algorithms with exponential speedup.
Challenges and Future Perspectives:
The development of reliable and scalable qubit technologies faces significant challenges. Decoherence, qubit initialization, gate errors, and interqubit connectivity pose hurdles to achieving fault-tolerant quantum computers. We discuss the ongoing efforts in qubit engineering, error correction, and novel qubit designs to address these challenges. Continued research and technological advancements are necessary to harness the full potential of qubits for practical quantum technologies.
Conclusion:
Quantum bits, or qubits, lie at the heart of quantum information processing, enabling powerful computational capabilities beyond the reach of classical systems. Their ability to exist in superposition and entanglement provides a unique foundation for quantum technology. Understanding the physical realizations, mathematical representation, properties, and challenges of qubits is essential for the development of scalable quantum computers and the realization of quantum applications in various fields.
References:
- Nielsen, M. A., & Chuang, I. L. (2010). Quantum Computation and Quantum Information. Cambridge University Press.
- Preskill, J. (2018). Quantum Computing in the NISQ era and beyond. Quantum, 2, 79.
- Devoret, M. H., & Schoelkopf, R. J. (2013). Superconducting circuits for quantum information: an outlook. Science, 339(6124), 1169-1174.
- Monroe, C., & Kim, J. (2013). Scaling the Ion Trap Quantum Processor. Science, 339(6124), 1164-1169.
- Awschalom, D. D., et al. (2018). Quantum Spintronics: Engineering and Manipulating Atom-Like Spins in Semiconductors. Science, 339(6124), 1174-1180.